Ideal for Aortic Tissue Homogenization
Do you spend lots of time and effort homogenizing aortic tissue samples? The Bullet Blender® tissue homogenizer delivers high quality and superior yields. No other homogenizer comes close to delivering the Bullet Blender’s winning combination of top-quality performance and budget-friendly affordability. See below for a aortic tissue homogenization protocol.
Save Time, Effort and Get Superior Results with
The Bullet Blender Homogenizer
Consistent and High Yield Results
Run up to 24 samples at the same time under microprocessor-controlled conditions, ensuring experimental reproducibility and high yield. Process samples from 10mg or less up to 3.5g.No Cross Contamination
No part of the Bullet Blender ever touches the tissue – the sample tubes are kept closed during homogenization. There are no probes to clean between samples.Samples Stay Cool
The Bullet Blenders’ innovative and elegant design provides convective cooling of the samples, so they do not heat up more than several degrees. In fact, our Gold+ models hold the sample temperature to about 4ºC.Easy and Convenient to Use
Just place beads and buffer along with your tissue sample in standard tubes, load tubes directly in the Bullet Blender, select time and speed, and press start.Risk Free Purchase
Thousands of peer-reviewed journal articles attest to the consistency and quality of the Bullet Blender homogenizer. We offer a 2 year warranty, extendable to 4 years, because our Bullet Blenders are reliable and last for many years.Aortic Tissue Homogenization Protocol
Sample size |
See the Protocol |
microcentrifuge tube model (up to 300 mg) | Small aortic samples |
5mL tube model (100mg - 1g) | Medium aortic samples |
What Else Can You Homogenize? Tough or Soft, No Problem!Â
The Bullet Blender can process a wide range of samples including organ tissue, cell culture, plant tissue, and small organisms. You can homogenize samples as tough as mouse femur or for gentle applications such as tissue dissociation or organelle isolation.
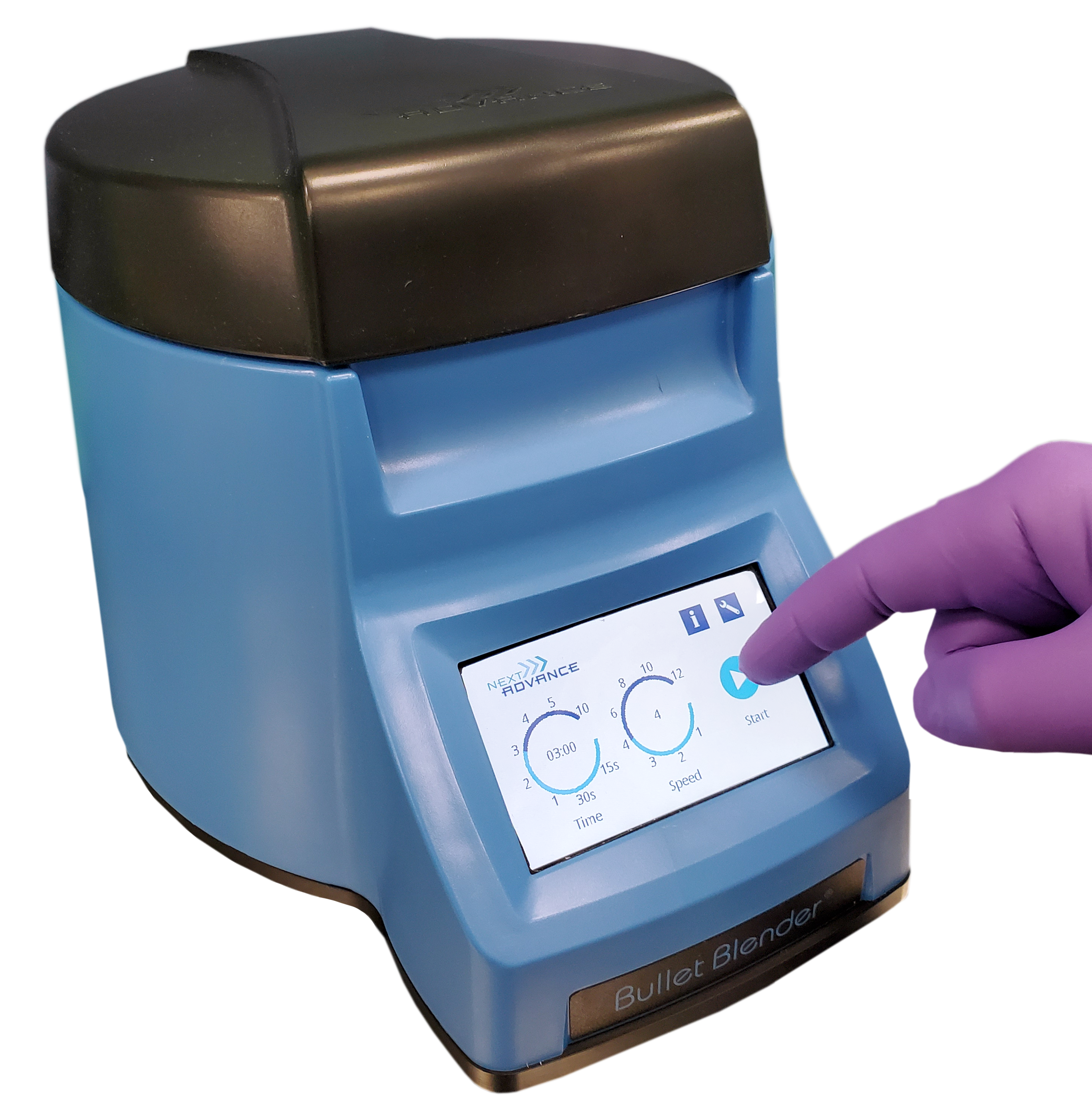
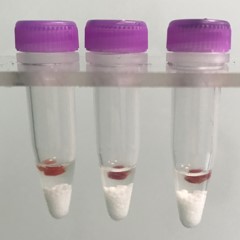
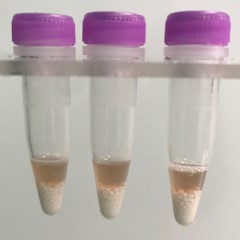
Aortic tissue pieces (on beads in upper photo) are completely homogenized into the buffer (darker in lower photo).Â
Want more guidance? Need a quote? Contact us:
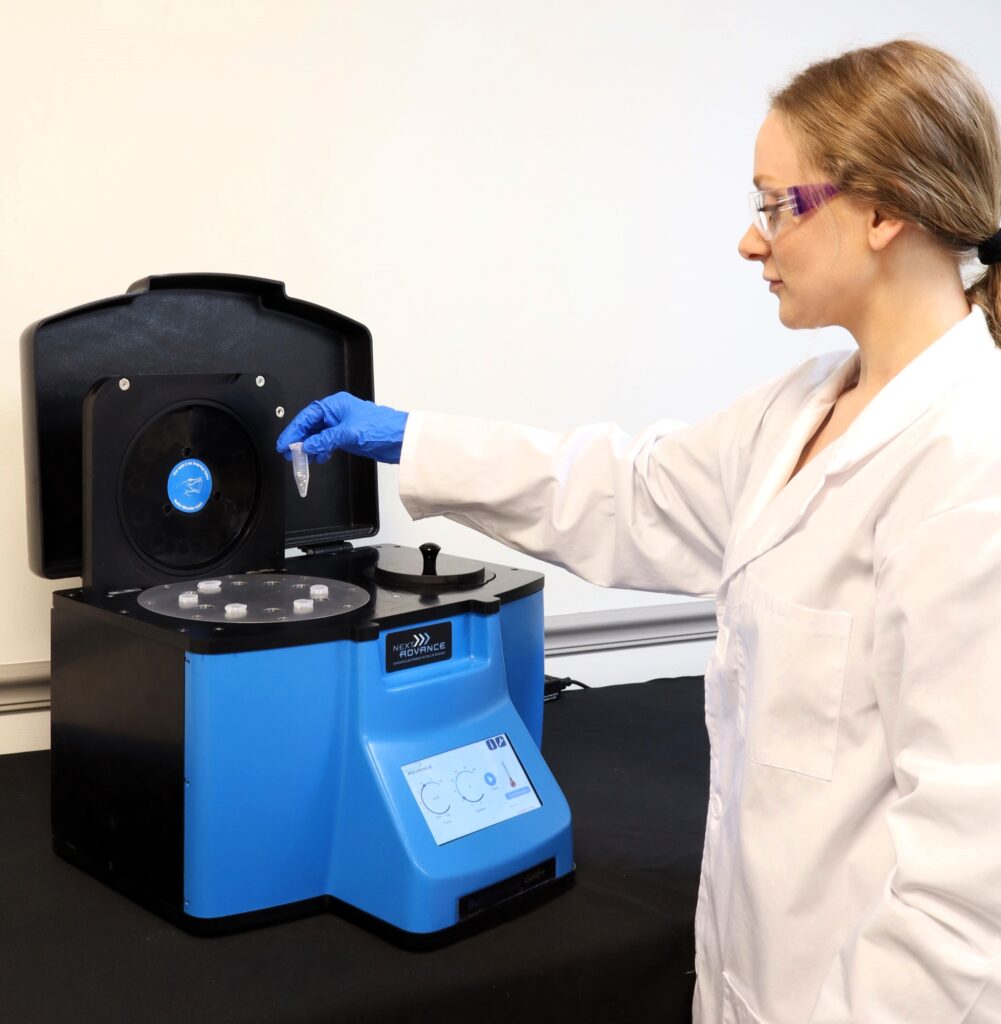
Bullet Blender Models
Select Publications using the Bullet Blender to Homogenize Aortic Tissue
2474232
aorta
1
apa
50
date
desc
3070
https://www.nextadvance.com/wp-content/plugins/zotpress/
%7B%22status%22%3A%22success%22%2C%22updateneeded%22%3Afalse%2C%22instance%22%3Afalse%2C%22meta%22%3A%7B%22request_last%22%3A0%2C%22request_next%22%3A0%2C%22used_cache%22%3Atrue%7D%2C%22data%22%3A%5B%7B%22key%22%3A%2298J6W6WE%22%2C%22library%22%3A%7B%22id%22%3A2474232%7D%2C%22meta%22%3A%7B%22creatorSummary%22%3A%22Papke%20et%20al.%22%2C%22parsedDate%22%3A%222015-10-15%22%2C%22numChildren%22%3A0%7D%2C%22bib%22%3A%22%26lt%3Bdiv%20class%3D%26quot%3Bcsl-bib-body%26quot%3B%20style%3D%26quot%3Bline-height%3A%202%3B%20padding-left%3A%201em%3B%20text-indent%3A-1em%3B%26quot%3B%26gt%3B%5Cn%20%20%26lt%3Bdiv%20class%3D%26quot%3Bcsl-entry%26quot%3B%26gt%3BPapke%2C%20C.%20L.%2C%20Tsunezumi%2C%20J.%2C%20Ringuette%2C%20L.-J.%2C%20Nagaoka%2C%20H.%2C%20Terajima%2C%20M.%2C%20Yamashiro%2C%20Y.%2C%20Urquhart%2C%20G.%2C%20Yamauchi%2C%20M.%2C%20Davis%2C%20E.%20C.%2C%20%26amp%3B%20Yanagisawa%2C%20H.%20%282015%29.%20Loss%20of%20fibulin-4%20disrupts%20collagen%20synthesis%20and%20maturation%3A%20implications%20for%20pathology%20resulting%20from%20%26lt%3Bi%26gt%3BEFEMP2%26lt%3B%5C%2Fi%26gt%3B%20mutations.%20%26lt%3Bi%26gt%3BHuman%20Molecular%20Genetics%26lt%3B%5C%2Fi%26gt%3B%2C%20%26lt%3Bi%26gt%3B24%26lt%3B%5C%2Fi%26gt%3B%2820%29%2C%205867%26%23x2013%3B5879.%20%26lt%3Ba%20class%3D%26%23039%3Bzp-DOIURL%26%23039%3B%20href%3D%26%23039%3Bhttps%3A%5C%2F%5C%2Fdoi.org%5C%2F10.1093%5C%2Fhmg%5C%2Fddv308%26%23039%3B%26gt%3Bhttps%3A%5C%2F%5C%2Fdoi.org%5C%2F10.1093%5C%2Fhmg%5C%2Fddv308%26lt%3B%5C%2Fa%26gt%3B%26lt%3B%5C%2Fdiv%26gt%3B%5Cn%26lt%3B%5C%2Fdiv%26gt%3B%22%2C%22data%22%3A%7B%22itemType%22%3A%22journalArticle%22%2C%22title%22%3A%22Loss%20of%20fibulin-4%20disrupts%20collagen%20synthesis%20and%20maturation%3A%20implications%20for%20pathology%20resulting%20from%20%3Ci%3EEFEMP2%3C%5C%2Fi%3E%20mutations%22%2C%22creators%22%3A%5B%7B%22creatorType%22%3A%22author%22%2C%22firstName%22%3A%22Christina%20L.%22%2C%22lastName%22%3A%22Papke%22%7D%2C%7B%22creatorType%22%3A%22author%22%2C%22firstName%22%3A%22Jun%22%2C%22lastName%22%3A%22Tsunezumi%22%7D%2C%7B%22creatorType%22%3A%22author%22%2C%22firstName%22%3A%22L%5Cu00e9a-Jeanne%22%2C%22lastName%22%3A%22Ringuette%22%7D%2C%7B%22creatorType%22%3A%22author%22%2C%22firstName%22%3A%22Hideaki%22%2C%22lastName%22%3A%22Nagaoka%22%7D%2C%7B%22creatorType%22%3A%22author%22%2C%22firstName%22%3A%22Masahiko%22%2C%22lastName%22%3A%22Terajima%22%7D%2C%7B%22creatorType%22%3A%22author%22%2C%22firstName%22%3A%22Yoshito%22%2C%22lastName%22%3A%22Yamashiro%22%7D%2C%7B%22creatorType%22%3A%22author%22%2C%22firstName%22%3A%22Greg%22%2C%22lastName%22%3A%22Urquhart%22%7D%2C%7B%22creatorType%22%3A%22author%22%2C%22firstName%22%3A%22Mitsuo%22%2C%22lastName%22%3A%22Yamauchi%22%7D%2C%7B%22creatorType%22%3A%22author%22%2C%22firstName%22%3A%22Elaine%20C.%22%2C%22lastName%22%3A%22Davis%22%7D%2C%7B%22creatorType%22%3A%22author%22%2C%22firstName%22%3A%22Hiromi%22%2C%22lastName%22%3A%22Yanagisawa%22%7D%5D%2C%22abstractNote%22%3A%22%22%2C%22date%22%3A%222015-10-15%22%2C%22language%22%3A%22en%22%2C%22DOI%22%3A%2210.1093%5C%2Fhmg%5C%2Fddv308%22%2C%22ISSN%22%3A%220964-6906%2C%201460-2083%22%2C%22url%22%3A%22http%3A%5C%2F%5C%2Fwww.hmg.oxfordjournals.org%5C%2Flookup%5C%2Fdoi%5C%2F10.1093%5C%2Fhmg%5C%2Fddv308%22%2C%22collections%22%3A%5B%22M2MNG549%22%5D%2C%22dateModified%22%3A%222016-01-04T21%3A53%3A31Z%22%7D%7D%2C%7B%22key%22%3A%22MVQMEHWE%22%2C%22library%22%3A%7B%22id%22%3A2474232%7D%2C%22meta%22%3A%7B%22creatorSummary%22%3A%22Reho%20et%20al.%22%2C%22parsedDate%22%3A%222015-05-01%22%2C%22numChildren%22%3A0%7D%2C%22bib%22%3A%22%26lt%3Bdiv%20class%3D%26quot%3Bcsl-bib-body%26quot%3B%20style%3D%26quot%3Bline-height%3A%202%3B%20padding-left%3A%201em%3B%20text-indent%3A-1em%3B%26quot%3B%26gt%3B%5Cn%20%20%26lt%3Bdiv%20class%3D%26quot%3Bcsl-entry%26quot%3B%26gt%3BReho%2C%20J.%20J.%2C%20Zheng%2C%20X.%2C%20Asico%2C%20L.%20D.%2C%20%26amp%3B%20Fisher%2C%20S.%20A.%20%282015%29.%20Redox%20signaling%20and%20splicing%20dependent%20change%20in%20myosin%20phosphatase%20underlie%20early%20versus%20late%20changes%20in%20NO%20vasodilator%20reserve%20in%20a%20mouse%20LPS%20model%20of%20sepsis.%20%26lt%3Bi%26gt%3BAmerican%20Journal%20of%20Physiology%20-%20Heart%20and%20Circulatory%20Physiology%26lt%3B%5C%2Fi%26gt%3B%2C%20%26lt%3Bi%26gt%3B308%26lt%3B%5C%2Fi%26gt%3B%289%29%2C%20H1039%26%23x2013%3BH1050.%20%26lt%3Ba%20class%3D%26%23039%3Bzp-DOIURL%26%23039%3B%20href%3D%26%23039%3Bhttps%3A%5C%2F%5C%2Fdoi.org%5C%2F10.1152%5C%2Fajpheart.00912.2014%26%23039%3B%26gt%3Bhttps%3A%5C%2F%5C%2Fdoi.org%5C%2F10.1152%5C%2Fajpheart.00912.2014%26lt%3B%5C%2Fa%26gt%3B%26lt%3B%5C%2Fdiv%26gt%3B%5Cn%26lt%3B%5C%2Fdiv%26gt%3B%22%2C%22data%22%3A%7B%22itemType%22%3A%22journalArticle%22%2C%22title%22%3A%22Redox%20signaling%20and%20splicing%20dependent%20change%20in%20myosin%20phosphatase%20underlie%20early%20versus%20late%20changes%20in%20NO%20vasodilator%20reserve%20in%20a%20mouse%20LPS%20model%20of%20sepsis%22%2C%22creators%22%3A%5B%7B%22creatorType%22%3A%22author%22%2C%22firstName%22%3A%22John%20J.%22%2C%22lastName%22%3A%22Reho%22%7D%2C%7B%22creatorType%22%3A%22author%22%2C%22firstName%22%3A%22Xiaoxu%22%2C%22lastName%22%3A%22Zheng%22%7D%2C%7B%22creatorType%22%3A%22author%22%2C%22firstName%22%3A%22Laureano%20D.%22%2C%22lastName%22%3A%22Asico%22%7D%2C%7B%22creatorType%22%3A%22author%22%2C%22firstName%22%3A%22Steven%20A.%22%2C%22lastName%22%3A%22Fisher%22%7D%5D%2C%22abstractNote%22%3A%22%22%2C%22date%22%3A%222015-05-01%22%2C%22language%22%3A%22en%22%2C%22DOI%22%3A%2210.1152%5C%2Fajpheart.00912.2014%22%2C%22ISSN%22%3A%220363-6135%2C%201522-1539%22%2C%22url%22%3A%22http%3A%5C%2F%5C%2Fajpheart.physiology.org%5C%2Flookup%5C%2Fdoi%5C%2F10.1152%5C%2Fajpheart.00912.2014%22%2C%22collections%22%3A%5B%22M2MNG549%22%5D%2C%22dateModified%22%3A%222015-08-19T17%3A18%3A08Z%22%7D%7D%2C%7B%22key%22%3A%22UTJKWCI4%22%2C%22library%22%3A%7B%22id%22%3A2474232%7D%2C%22meta%22%3A%7B%22creatorSummary%22%3A%22Rotllan%20et%20al.%22%2C%22parsedDate%22%3A%222015-02-01%22%2C%22numChildren%22%3A0%7D%2C%22bib%22%3A%22%26lt%3Bdiv%20class%3D%26quot%3Bcsl-bib-body%26quot%3B%20style%3D%26quot%3Bline-height%3A%202%3B%20padding-left%3A%201em%3B%20text-indent%3A-1em%3B%26quot%3B%26gt%3B%5Cn%20%20%26lt%3Bdiv%20class%3D%26quot%3Bcsl-entry%26quot%3B%26gt%3BRotllan%2C%20N.%2C%20Chamorro-Jorganes%2C%20A.%2C%20Araldi%2C%20E.%2C%20Wanschel%2C%20A.%20C.%2C%20Aryal%2C%20B.%2C%20Aranda%2C%20J.%20F.%2C%20Goedeke%2C%20L.%2C%20Salerno%2C%20A.%20G.%2C%20Ramirez%2C%20C.%20M.%2C%20Sessa%2C%20W.%20C.%2C%20Suarez%2C%20Y.%2C%20%26amp%3B%20Fernandez-Hernando%2C%20C.%20%282015%29.%20Hematopoietic%20Akt2%20deficiency%20attenuates%20the%20progression%20of%20atherosclerosis.%20%26lt%3Bi%26gt%3BThe%20FASEB%20Journal%26lt%3B%5C%2Fi%26gt%3B%2C%20%26lt%3Bi%26gt%3B29%26lt%3B%5C%2Fi%26gt%3B%282%29%2C%20597%26%23x2013%3B610.%20%26lt%3Ba%20class%3D%26%23039%3Bzp-DOIURL%26%23039%3B%20href%3D%26%23039%3Bhttps%3A%5C%2F%5C%2Fdoi.org%5C%2F10.1096%5C%2Ffj.14-262097%26%23039%3B%26gt%3Bhttps%3A%5C%2F%5C%2Fdoi.org%5C%2F10.1096%5C%2Ffj.14-262097%26lt%3B%5C%2Fa%26gt%3B%26lt%3B%5C%2Fdiv%26gt%3B%5Cn%26lt%3B%5C%2Fdiv%26gt%3B%22%2C%22data%22%3A%7B%22itemType%22%3A%22journalArticle%22%2C%22title%22%3A%22Hematopoietic%20Akt2%20deficiency%20attenuates%20the%20progression%20of%20atherosclerosis%22%2C%22creators%22%3A%5B%7B%22creatorType%22%3A%22author%22%2C%22firstName%22%3A%22N.%22%2C%22lastName%22%3A%22Rotllan%22%7D%2C%7B%22creatorType%22%3A%22author%22%2C%22firstName%22%3A%22A.%22%2C%22lastName%22%3A%22Chamorro-Jorganes%22%7D%2C%7B%22creatorType%22%3A%22author%22%2C%22firstName%22%3A%22E.%22%2C%22lastName%22%3A%22Araldi%22%7D%2C%7B%22creatorType%22%3A%22author%22%2C%22firstName%22%3A%22A.%20C.%22%2C%22lastName%22%3A%22Wanschel%22%7D%2C%7B%22creatorType%22%3A%22author%22%2C%22firstName%22%3A%22B.%22%2C%22lastName%22%3A%22Aryal%22%7D%2C%7B%22creatorType%22%3A%22author%22%2C%22firstName%22%3A%22J.%20F.%22%2C%22lastName%22%3A%22Aranda%22%7D%2C%7B%22creatorType%22%3A%22author%22%2C%22firstName%22%3A%22L.%22%2C%22lastName%22%3A%22Goedeke%22%7D%2C%7B%22creatorType%22%3A%22author%22%2C%22firstName%22%3A%22A.%20G.%22%2C%22lastName%22%3A%22Salerno%22%7D%2C%7B%22creatorType%22%3A%22author%22%2C%22firstName%22%3A%22C.%20M.%22%2C%22lastName%22%3A%22Ramirez%22%7D%2C%7B%22creatorType%22%3A%22author%22%2C%22firstName%22%3A%22W.%20C.%22%2C%22lastName%22%3A%22Sessa%22%7D%2C%7B%22creatorType%22%3A%22author%22%2C%22firstName%22%3A%22Y.%22%2C%22lastName%22%3A%22Suarez%22%7D%2C%7B%22creatorType%22%3A%22author%22%2C%22firstName%22%3A%22C.%22%2C%22lastName%22%3A%22Fernandez-Hernando%22%7D%5D%2C%22abstractNote%22%3A%22%22%2C%22date%22%3A%222015-02-01%22%2C%22language%22%3A%22en%22%2C%22DOI%22%3A%2210.1096%5C%2Ffj.14-262097%22%2C%22ISSN%22%3A%220892-6638%2C%201530-6860%22%2C%22url%22%3A%22http%3A%5C%2F%5C%2Fwww.fasebj.org%5C%2Fcgi%5C%2Fdoi%5C%2F10.1096%5C%2Ffj.14-262097%22%2C%22collections%22%3A%5B%22M2MNG549%22%5D%2C%22dateModified%22%3A%222015-12-31T20%3A51%3A47Z%22%7D%7D%2C%7B%22key%22%3A%22U2E8I68S%22%2C%22library%22%3A%7B%22id%22%3A2474232%7D%2C%22meta%22%3A%7B%22creatorSummary%22%3A%22Carlstr%5Cu00f6m%20et%20al.%22%2C%22parsedDate%22%3A%222015%22%2C%22numChildren%22%3A0%7D%2C%22bib%22%3A%22%26lt%3Bdiv%20class%3D%26quot%3Bcsl-bib-body%26quot%3B%20style%3D%26quot%3Bline-height%3A%202%3B%20padding-left%3A%201em%3B%20text-indent%3A-1em%3B%26quot%3B%26gt%3B%5Cn%20%20%26lt%3Bdiv%20class%3D%26quot%3Bcsl-entry%26quot%3B%26gt%3BCarlstr%26%23xF6%3Bm%2C%20M.%2C%20Liu%2C%20M.%2C%20Yang%2C%20T.%2C%20Zollbrecht%2C%20C.%2C%20Huang%2C%20L.%2C%20Peleli%2C%20M.%2C%20Borniquel%2C%20S.%2C%20Kishikawa%2C%20H.%2C%20Hezel%2C%20M.%2C%20Persson%2C%20A.%20E.%20G.%2C%20Weitzberg%2C%20E.%2C%20%26amp%3B%20Lundberg%2C%20J.%20O.%20%282015%29.%20Cross-talk%20Between%20Nitrate-Nitrite-NO%20and%20NO%20Synthase%20Pathways%20in%20Control%20of%20Vascular%20NO%20Homeostasis.%20%26lt%3Bi%26gt%3BAntioxidants%20%26amp%3B%20Redox%20Signaling%26lt%3B%5C%2Fi%26gt%3B%2C%20%26lt%3Bi%26gt%3B23%26lt%3B%5C%2Fi%26gt%3B%284%29%2C%20295%26%23x2013%3B306.%20%26lt%3Ba%20class%3D%26%23039%3Bzp-DOIURL%26%23039%3B%20href%3D%26%23039%3Bhttps%3A%5C%2F%5C%2Fdoi.org%5C%2F10.1089%5C%2Fars.2013.5481%26%23039%3B%26gt%3Bhttps%3A%5C%2F%5C%2Fdoi.org%5C%2F10.1089%5C%2Fars.2013.5481%26lt%3B%5C%2Fa%26gt%3B%26lt%3B%5C%2Fdiv%26gt%3B%5Cn%26lt%3B%5C%2Fdiv%26gt%3B%22%2C%22data%22%3A%7B%22itemType%22%3A%22journalArticle%22%2C%22title%22%3A%22Cross-talk%20Between%20Nitrate-Nitrite-NO%20and%20NO%20Synthase%20Pathways%20in%20Control%20of%20Vascular%20NO%20Homeostasis%22%2C%22creators%22%3A%5B%7B%22creatorType%22%3A%22author%22%2C%22firstName%22%3A%22Mattias%22%2C%22lastName%22%3A%22Carlstr%5Cu00f6m%22%7D%2C%7B%22creatorType%22%3A%22author%22%2C%22firstName%22%3A%22Ming%22%2C%22lastName%22%3A%22Liu%22%7D%2C%7B%22creatorType%22%3A%22author%22%2C%22firstName%22%3A%22Ting%22%2C%22lastName%22%3A%22Yang%22%7D%2C%7B%22creatorType%22%3A%22author%22%2C%22firstName%22%3A%22Christa%22%2C%22lastName%22%3A%22Zollbrecht%22%7D%2C%7B%22creatorType%22%3A%22author%22%2C%22firstName%22%3A%22Liyue%22%2C%22lastName%22%3A%22Huang%22%7D%2C%7B%22creatorType%22%3A%22author%22%2C%22firstName%22%3A%22Maria%22%2C%22lastName%22%3A%22Peleli%22%7D%2C%7B%22creatorType%22%3A%22author%22%2C%22firstName%22%3A%22Sara%22%2C%22lastName%22%3A%22Borniquel%22%7D%2C%7B%22creatorType%22%3A%22author%22%2C%22firstName%22%3A%22Hiroaki%22%2C%22lastName%22%3A%22Kishikawa%22%7D%2C%7B%22creatorType%22%3A%22author%22%2C%22firstName%22%3A%22Michael%22%2C%22lastName%22%3A%22Hezel%22%7D%2C%7B%22creatorType%22%3A%22author%22%2C%22firstName%22%3A%22A.%20Erik%20G.%22%2C%22lastName%22%3A%22Persson%22%7D%2C%7B%22creatorType%22%3A%22author%22%2C%22firstName%22%3A%22Eddie%22%2C%22lastName%22%3A%22Weitzberg%22%7D%2C%7B%22creatorType%22%3A%22author%22%2C%22firstName%22%3A%22Jon%20O.%22%2C%22lastName%22%3A%22Lundberg%22%7D%5D%2C%22abstractNote%22%3A%22%22%2C%22date%22%3A%2208%5C%2F2015%22%2C%22language%22%3A%22en%22%2C%22DOI%22%3A%2210.1089%5C%2Fars.2013.5481%22%2C%22ISSN%22%3A%221523-0864%2C%201557-7716%22%2C%22url%22%3A%22http%3A%5C%2F%5C%2Fonline.liebertpub.com%5C%2Fdoi%5C%2F10.1089%5C%2Fars.2013.5481%22%2C%22collections%22%3A%5B%22M2MNG549%22%5D%2C%22dateModified%22%3A%222015-08-05T17%3A48%3A27Z%22%7D%7D%2C%7B%22key%22%3A%22T98EXXJJ%22%2C%22library%22%3A%7B%22id%22%3A2474232%7D%2C%22meta%22%3A%7B%22creatorSummary%22%3A%22Gao%20et%20al.%22%2C%22parsedDate%22%3A%222013-03-15%22%2C%22numChildren%22%3A0%7D%2C%22bib%22%3A%22%26lt%3Bdiv%20class%3D%26quot%3Bcsl-bib-body%26quot%3B%20style%3D%26quot%3Bline-height%3A%202%3B%20padding-left%3A%201em%3B%20text-indent%3A-1em%3B%26quot%3B%26gt%3B%5Cn%20%20%26lt%3Bdiv%20class%3D%26quot%3Bcsl-entry%26quot%3B%26gt%3BGao%2C%20N.%2C%20Huang%2C%20J.%2C%20He%2C%20W.%2C%20Zhu%2C%20M.%2C%20Kamm%2C%20K.%20E.%2C%20%26amp%3B%20Stull%2C%20J.%20T.%20%282013%29.%20Signaling%20through%20Myosin%20Light%20Chain%20Kinase%20in%20Smooth%20Muscles.%20%26lt%3Bi%26gt%3BJournal%20of%20Biological%20Chemistry%26lt%3B%5C%2Fi%26gt%3B%2C%20%26lt%3Bi%26gt%3B288%26lt%3B%5C%2Fi%26gt%3B%2811%29%2C%207596%26%23x2013%3B7605.%20%26lt%3Ba%20class%3D%26%23039%3Bzp-DOIURL%26%23039%3B%20href%3D%26%23039%3Bhttps%3A%5C%2F%5C%2Fdoi.org%5C%2F10.1074%5C%2Fjbc.M112.427112%26%23039%3B%26gt%3Bhttps%3A%5C%2F%5C%2Fdoi.org%5C%2F10.1074%5C%2Fjbc.M112.427112%26lt%3B%5C%2Fa%26gt%3B%26lt%3B%5C%2Fdiv%26gt%3B%5Cn%26lt%3B%5C%2Fdiv%26gt%3B%22%2C%22data%22%3A%7B%22itemType%22%3A%22journalArticle%22%2C%22title%22%3A%22Signaling%20through%20Myosin%20Light%20Chain%20Kinase%20in%20Smooth%20Muscles%22%2C%22creators%22%3A%5B%7B%22creatorType%22%3A%22author%22%2C%22firstName%22%3A%22N.%22%2C%22lastName%22%3A%22Gao%22%7D%2C%7B%22creatorType%22%3A%22author%22%2C%22firstName%22%3A%22J.%22%2C%22lastName%22%3A%22Huang%22%7D%2C%7B%22creatorType%22%3A%22author%22%2C%22firstName%22%3A%22W.%22%2C%22lastName%22%3A%22He%22%7D%2C%7B%22creatorType%22%3A%22author%22%2C%22firstName%22%3A%22M.%22%2C%22lastName%22%3A%22Zhu%22%7D%2C%7B%22creatorType%22%3A%22author%22%2C%22firstName%22%3A%22K.%20E.%22%2C%22lastName%22%3A%22Kamm%22%7D%2C%7B%22creatorType%22%3A%22author%22%2C%22firstName%22%3A%22J.%20T.%22%2C%22lastName%22%3A%22Stull%22%7D%5D%2C%22abstractNote%22%3A%22%22%2C%22date%22%3A%222013-03-15%22%2C%22language%22%3A%22en%22%2C%22DOI%22%3A%2210.1074%5C%2Fjbc.M112.427112%22%2C%22ISSN%22%3A%220021-9258%2C%201083-351X%22%2C%22url%22%3A%22http%3A%5C%2F%5C%2Fwww.jbc.org%5C%2Fcgi%5C%2Fdoi%5C%2F10.1074%5C%2Fjbc.M112.427112%22%2C%22collections%22%3A%5B%22M2MNG549%22%5D%2C%22dateModified%22%3A%222015-07-13T16%3A38%3A44Z%22%7D%7D%2C%7B%22key%22%3A%22G9GQZHQD%22%2C%22library%22%3A%7B%22id%22%3A2474232%7D%2C%22meta%22%3A%7B%22creatorSummary%22%3A%22Kuang%20et%20al.%22%2C%22parsedDate%22%3A%222012-05-25%22%2C%22numChildren%22%3A0%7D%2C%22bib%22%3A%22%26lt%3Bdiv%20class%3D%26quot%3Bcsl-bib-body%26quot%3B%20style%3D%26quot%3Bline-height%3A%202%3B%20padding-left%3A%201em%3B%20text-indent%3A-1em%3B%26quot%3B%26gt%3B%5Cn%20%20%26lt%3Bdiv%20class%3D%26quot%3Bcsl-entry%26quot%3B%26gt%3BKuang%2C%20S.-Q.%2C%20Kwartler%2C%20C.%20S.%2C%20Byanova%2C%20K.%20L.%2C%20Pham%2C%20J.%2C%20Gong%2C%20L.%2C%20Prakash%2C%20S.%20K.%2C%20Huang%2C%20J.%2C%20Kamm%2C%20K.%20E.%2C%20Stull%2C%20J.%20T.%2C%20Sweeney%2C%20H.%20L.%2C%20%26amp%3B%20Milewicz%2C%20D.%20M.%20%282012%29.%20Rare%2C%20Nonsynonymous%20Variant%20in%20the%20Smooth%20Muscle-Specific%20Isoform%20of%20Myosin%20Heavy%20Chain%2C%20MYH11%2C%20R247C%2C%20Alters%20Force%20Generation%20in%20the%20Aorta%20and%20Phenotype%20of%20Smooth%20Muscle%20Cells.%20%26lt%3Bi%26gt%3BCirculation%20Research%26lt%3B%5C%2Fi%26gt%3B%2C%20%26lt%3Bi%26gt%3B110%26lt%3B%5C%2Fi%26gt%3B%2811%29%2C%201411%26%23x2013%3B1422.%20%26lt%3Ba%20class%3D%26%23039%3Bzp-DOIURL%26%23039%3B%20href%3D%26%23039%3Bhttps%3A%5C%2F%5C%2Fdoi.org%5C%2F10.1161%5C%2FCIRCRESAHA.111.261743%26%23039%3B%26gt%3Bhttps%3A%5C%2F%5C%2Fdoi.org%5C%2F10.1161%5C%2FCIRCRESAHA.111.261743%26lt%3B%5C%2Fa%26gt%3B%26lt%3B%5C%2Fdiv%26gt%3B%5Cn%26lt%3B%5C%2Fdiv%26gt%3B%22%2C%22data%22%3A%7B%22itemType%22%3A%22journalArticle%22%2C%22title%22%3A%22Rare%2C%20Nonsynonymous%20Variant%20in%20the%20Smooth%20Muscle-Specific%20Isoform%20of%20Myosin%20Heavy%20Chain%2C%20MYH11%2C%20R247C%2C%20Alters%20Force%20Generation%20in%20the%20Aorta%20and%20Phenotype%20of%20Smooth%20Muscle%20Cells%22%2C%22creators%22%3A%5B%7B%22creatorType%22%3A%22author%22%2C%22firstName%22%3A%22S.-Q.%22%2C%22lastName%22%3A%22Kuang%22%7D%2C%7B%22creatorType%22%3A%22author%22%2C%22firstName%22%3A%22C.%20S.%22%2C%22lastName%22%3A%22Kwartler%22%7D%2C%7B%22creatorType%22%3A%22author%22%2C%22firstName%22%3A%22K.%20L.%22%2C%22lastName%22%3A%22Byanova%22%7D%2C%7B%22creatorType%22%3A%22author%22%2C%22firstName%22%3A%22J.%22%2C%22lastName%22%3A%22Pham%22%7D%2C%7B%22creatorType%22%3A%22author%22%2C%22firstName%22%3A%22L.%22%2C%22lastName%22%3A%22Gong%22%7D%2C%7B%22creatorType%22%3A%22author%22%2C%22firstName%22%3A%22S.%20K.%22%2C%22lastName%22%3A%22Prakash%22%7D%2C%7B%22creatorType%22%3A%22author%22%2C%22firstName%22%3A%22J.%22%2C%22lastName%22%3A%22Huang%22%7D%2C%7B%22creatorType%22%3A%22author%22%2C%22firstName%22%3A%22K.%20E.%22%2C%22lastName%22%3A%22Kamm%22%7D%2C%7B%22creatorType%22%3A%22author%22%2C%22firstName%22%3A%22J.%20T.%22%2C%22lastName%22%3A%22Stull%22%7D%2C%7B%22creatorType%22%3A%22author%22%2C%22firstName%22%3A%22H.%20L.%22%2C%22lastName%22%3A%22Sweeney%22%7D%2C%7B%22creatorType%22%3A%22author%22%2C%22firstName%22%3A%22D.%20M.%22%2C%22lastName%22%3A%22Milewicz%22%7D%5D%2C%22abstractNote%22%3A%22%22%2C%22date%22%3A%222012-05-25%22%2C%22language%22%3A%22en%22%2C%22DOI%22%3A%2210.1161%5C%2FCIRCRESAHA.111.261743%22%2C%22ISSN%22%3A%220009-7330%2C%201524-4571%22%2C%22url%22%3A%22http%3A%5C%2F%5C%2Fcircres.ahajournals.org%5C%2Fcgi%5C%2Fdoi%5C%2F10.1161%5C%2FCIRCRESAHA.111.261743%22%2C%22collections%22%3A%5B%22M2MNG549%22%5D%2C%22dateModified%22%3A%222015-07-13T16%3A48%3A51Z%22%7D%7D%2C%7B%22key%22%3A%22INDQTUME%22%2C%22library%22%3A%7B%22id%22%3A2474232%7D%2C%22meta%22%3A%7B%22creatorSummary%22%3A%22Fox%20et%20al.%22%2C%22parsedDate%22%3A%222012%22%2C%22numChildren%22%3A0%7D%2C%22bib%22%3A%22%26lt%3Bdiv%20class%3D%26quot%3Bcsl-bib-body%26quot%3B%20style%3D%26quot%3Bline-height%3A%202%3B%20padding-left%3A%201em%3B%20text-indent%3A-1em%3B%26quot%3B%26gt%3B%5Cn%20%20%26lt%3Bdiv%20class%3D%26quot%3Bcsl-entry%26quot%3B%26gt%3BFox%2C%20K.%20A.%2C%20Longo%2C%20M.%2C%20Tamayo%2C%20E.%2C%20Gamble%2C%20P.%2C%20Makhlouf%2C%20M.%2C%20Mateus%2C%20J.%20F.%2C%20%26amp%3B%20Saade%2C%20G.%20R.%20%282012%29.%20Sex-specific%20effects%20of%20nicotine%20exposure%20on%20developmental%20programming%20of%20blood%20pressure%20and%20vascular%20reactivity%20in%20the%20C57Bl%5C%2F6J%20mouse.%20%26lt%3Bi%26gt%3BAmerican%20Journal%20of%20Obstetrics%20and%20Gynecology%26lt%3B%5C%2Fi%26gt%3B%2C%20%26lt%3Bi%26gt%3B207%26lt%3B%5C%2Fi%26gt%3B%283%29%2C%20208.e1-208.e9.%20%26lt%3Ba%20class%3D%26%23039%3Bzp-DOIURL%26%23039%3B%20href%3D%26%23039%3Bhttps%3A%5C%2F%5C%2Fdoi.org%5C%2F10.1016%5C%2Fj.ajog.2012.06.021%26%23039%3B%26gt%3Bhttps%3A%5C%2F%5C%2Fdoi.org%5C%2F10.1016%5C%2Fj.ajog.2012.06.021%26lt%3B%5C%2Fa%26gt%3B%26lt%3B%5C%2Fdiv%26gt%3B%5Cn%26lt%3B%5C%2Fdiv%26gt%3B%22%2C%22data%22%3A%7B%22itemType%22%3A%22journalArticle%22%2C%22title%22%3A%22Sex-specific%20effects%20of%20nicotine%20exposure%20on%20developmental%20programming%20of%20blood%20pressure%20and%20vascular%20reactivity%20in%20the%20C57Bl%5C%2F6J%20mouse%22%2C%22creators%22%3A%5B%7B%22creatorType%22%3A%22author%22%2C%22firstName%22%3A%22Karin%20A.%22%2C%22lastName%22%3A%22Fox%22%7D%2C%7B%22creatorType%22%3A%22author%22%2C%22firstName%22%3A%22Monica%22%2C%22lastName%22%3A%22Longo%22%7D%2C%7B%22creatorType%22%3A%22author%22%2C%22firstName%22%3A%22Esther%22%2C%22lastName%22%3A%22Tamayo%22%7D%2C%7B%22creatorType%22%3A%22author%22%2C%22firstName%22%3A%22Phyllis%22%2C%22lastName%22%3A%22Gamble%22%7D%2C%7B%22creatorType%22%3A%22author%22%2C%22firstName%22%3A%22Michel%22%2C%22lastName%22%3A%22Makhlouf%22%7D%2C%7B%22creatorType%22%3A%22author%22%2C%22firstName%22%3A%22Julio%20F.%22%2C%22lastName%22%3A%22Mateus%22%7D%2C%7B%22creatorType%22%3A%22author%22%2C%22firstName%22%3A%22George%20R.%22%2C%22lastName%22%3A%22Saade%22%7D%5D%2C%22abstractNote%22%3A%22%22%2C%22date%22%3A%2209%5C%2F2012%22%2C%22language%22%3A%22en%22%2C%22DOI%22%3A%2210.1016%5C%2Fj.ajog.2012.06.021%22%2C%22ISSN%22%3A%2200029378%22%2C%22url%22%3A%22http%3A%5C%2F%5C%2Flinkinghub.elsevier.com%5C%2Fretrieve%5C%2Fpii%5C%2FS0002937812006229%22%2C%22collections%22%3A%5B%22M2MNG549%22%5D%2C%22dateModified%22%3A%222015-07-20T16%3A35%3A26Z%22%7D%7D%2C%7B%22key%22%3A%229D4N5EFC%22%2C%22library%22%3A%7B%22id%22%3A2474232%7D%2C%22meta%22%3A%7B%22creatorSummary%22%3A%22Shai%20et%20al.%22%2C%22parsedDate%22%3A%222011-05-01%22%2C%22numChildren%22%3A0%7D%2C%22bib%22%3A%22%26lt%3Bdiv%20class%3D%26quot%3Bcsl-bib-body%26quot%3B%20style%3D%26quot%3Bline-height%3A%202%3B%20padding-left%3A%201em%3B%20text-indent%3A-1em%3B%26quot%3B%26gt%3B%5Cn%20%20%26lt%3Bdiv%20class%3D%26quot%3Bcsl-entry%26quot%3B%26gt%3BShai%2C%20S.-Y.%2C%20Sukhanov%2C%20S.%2C%20Higashi%2C%20Y.%2C%20Vaughn%2C%20C.%2C%20Rosen%2C%20C.%20J.%2C%20%26amp%3B%20Delafontaine%2C%20P.%20%282011%29.%20Low%20circulating%20insulin-like%20growth%20factor%20I%20increases%20atherosclerosis%20in%20ApoE-deficient%20mice.%20%26lt%3Bi%26gt%3BAJP%3A%20Heart%20and%20Circulatory%20Physiology%26lt%3B%5C%2Fi%26gt%3B%2C%20%26lt%3Bi%26gt%3B300%26lt%3B%5C%2Fi%26gt%3B%285%29%2C%20H1898%26%23x2013%3BH1906.%20%26lt%3Ba%20class%3D%26%23039%3Bzp-DOIURL%26%23039%3B%20href%3D%26%23039%3Bhttps%3A%5C%2F%5C%2Fdoi.org%5C%2F10.1152%5C%2Fajpheart.01081.2010%26%23039%3B%26gt%3Bhttps%3A%5C%2F%5C%2Fdoi.org%5C%2F10.1152%5C%2Fajpheart.01081.2010%26lt%3B%5C%2Fa%26gt%3B%26lt%3B%5C%2Fdiv%26gt%3B%5Cn%26lt%3B%5C%2Fdiv%26gt%3B%22%2C%22data%22%3A%7B%22itemType%22%3A%22journalArticle%22%2C%22title%22%3A%22Low%20circulating%20insulin-like%20growth%20factor%20I%20increases%20atherosclerosis%20in%20ApoE-deficient%20mice%22%2C%22creators%22%3A%5B%7B%22creatorType%22%3A%22author%22%2C%22firstName%22%3A%22S.-Y.%22%2C%22lastName%22%3A%22Shai%22%7D%2C%7B%22creatorType%22%3A%22author%22%2C%22firstName%22%3A%22S.%22%2C%22lastName%22%3A%22Sukhanov%22%7D%2C%7B%22creatorType%22%3A%22author%22%2C%22firstName%22%3A%22Y.%22%2C%22lastName%22%3A%22Higashi%22%7D%2C%7B%22creatorType%22%3A%22author%22%2C%22firstName%22%3A%22C.%22%2C%22lastName%22%3A%22Vaughn%22%7D%2C%7B%22creatorType%22%3A%22author%22%2C%22firstName%22%3A%22C.%20J.%22%2C%22lastName%22%3A%22Rosen%22%7D%2C%7B%22creatorType%22%3A%22author%22%2C%22firstName%22%3A%22P.%22%2C%22lastName%22%3A%22Delafontaine%22%7D%5D%2C%22abstractNote%22%3A%22%22%2C%22date%22%3A%222011-05-01%22%2C%22language%22%3A%22en%22%2C%22DOI%22%3A%2210.1152%5C%2Fajpheart.01081.2010%22%2C%22ISSN%22%3A%220363-6135%2C%201522-1539%22%2C%22url%22%3A%22http%3A%5C%2F%5C%2Fajpheart.physiology.org%5C%2Fcgi%5C%2Fdoi%5C%2F10.1152%5C%2Fajpheart.01081.2010%22%2C%22collections%22%3A%5B%22M2MNG549%22%5D%2C%22dateModified%22%3A%222015-07-22T14%3A09%3A45Z%22%7D%7D%2C%7B%22key%22%3A%22S32T26M4%22%2C%22library%22%3A%7B%22id%22%3A2474232%7D%2C%22meta%22%3A%7B%22creatorSummary%22%3A%22Fox%20et%20al.%22%2C%22parsedDate%22%3A%222011%22%2C%22numChildren%22%3A0%7D%2C%22bib%22%3A%22%26lt%3Bdiv%20class%3D%26quot%3Bcsl-bib-body%26quot%3B%20style%3D%26quot%3Bline-height%3A%202%3B%20padding-left%3A%201em%3B%20text-indent%3A-1em%3B%26quot%3B%26gt%3B%5Cn%20%20%26lt%3Bdiv%20class%3D%26quot%3Bcsl-entry%26quot%3B%26gt%3BFox%2C%20K.%20A.%2C%20Longo%2C%20M.%2C%20Tamayo%2C%20E.%2C%20Kechichian%2C%20T.%2C%20Bytautiene%2C%20E.%2C%20Hankins%2C%20G.%20D.%20V.%2C%20Saade%2C%20G.%20R.%2C%20%26amp%3B%20Costantine%2C%20M.%20M.%20%282011%29.%20Effects%20of%20pravastatin%20on%20mediators%20of%20vascular%20function%20in%20a%20mouse%20model%20of%20soluble%20Fms-like%20tyrosine%20kinase-1%26%23x2013%3Binduced%20preeclampsia.%20%26lt%3Bi%26gt%3BAmerican%20Journal%20of%20Obstetrics%20and%20Gynecology%26lt%3B%5C%2Fi%26gt%3B%2C%20%26lt%3Bi%26gt%3B205%26lt%3B%5C%2Fi%26gt%3B%284%29%2C%20366.e1-366.e5.%20%26lt%3Ba%20class%3D%26%23039%3Bzp-DOIURL%26%23039%3B%20href%3D%26%23039%3Bhttps%3A%5C%2F%5C%2Fdoi.org%5C%2F10.1016%5C%2Fj.ajog.2011.06.083%26%23039%3B%26gt%3Bhttps%3A%5C%2F%5C%2Fdoi.org%5C%2F10.1016%5C%2Fj.ajog.2011.06.083%26lt%3B%5C%2Fa%26gt%3B%26lt%3B%5C%2Fdiv%26gt%3B%5Cn%26lt%3B%5C%2Fdiv%26gt%3B%22%2C%22data%22%3A%7B%22itemType%22%3A%22journalArticle%22%2C%22title%22%3A%22Effects%20of%20pravastatin%20on%20mediators%20of%20vascular%20function%20in%20a%20mouse%20model%20of%20soluble%20Fms-like%20tyrosine%20kinase-1%5Cu2013induced%20preeclampsia%22%2C%22creators%22%3A%5B%7B%22creatorType%22%3A%22author%22%2C%22firstName%22%3A%22Karin%20A.%22%2C%22lastName%22%3A%22Fox%22%7D%2C%7B%22creatorType%22%3A%22author%22%2C%22firstName%22%3A%22Monica%22%2C%22lastName%22%3A%22Longo%22%7D%2C%7B%22creatorType%22%3A%22author%22%2C%22firstName%22%3A%22Esther%22%2C%22lastName%22%3A%22Tamayo%22%7D%2C%7B%22creatorType%22%3A%22author%22%2C%22firstName%22%3A%22Talar%22%2C%22lastName%22%3A%22Kechichian%22%7D%2C%7B%22creatorType%22%3A%22author%22%2C%22firstName%22%3A%22Egle%22%2C%22lastName%22%3A%22Bytautiene%22%7D%2C%7B%22creatorType%22%3A%22author%22%2C%22firstName%22%3A%22Gary%20D.V.%22%2C%22lastName%22%3A%22Hankins%22%7D%2C%7B%22creatorType%22%3A%22author%22%2C%22firstName%22%3A%22George%20R.%22%2C%22lastName%22%3A%22Saade%22%7D%2C%7B%22creatorType%22%3A%22author%22%2C%22firstName%22%3A%22Maged%20M.%22%2C%22lastName%22%3A%22Costantine%22%7D%5D%2C%22abstractNote%22%3A%22%22%2C%22date%22%3A%2210%5C%2F2011%22%2C%22language%22%3A%22en%22%2C%22DOI%22%3A%2210.1016%5C%2Fj.ajog.2011.06.083%22%2C%22ISSN%22%3A%2200029378%22%2C%22url%22%3A%22http%3A%5C%2F%5C%2Flinkinghub.elsevier.com%5C%2Fretrieve%5C%2Fpii%5C%2FS0002937811008313%22%2C%22collections%22%3A%5B%22M2MNG549%22%5D%2C%22dateModified%22%3A%222015-07-23T16%3A58%3A26Z%22%7D%7D%2C%7B%22key%22%3A%22EVHFA7JI%22%2C%22library%22%3A%7B%22id%22%3A2474232%7D%2C%22meta%22%3A%7B%22creatorSummary%22%3A%22Rayner%20et%20al.%22%2C%22parsedDate%22%3A%222010-06-18%22%2C%22numChildren%22%3A0%7D%2C%22bib%22%3A%22%26lt%3Bdiv%20class%3D%26quot%3Bcsl-bib-body%26quot%3B%20style%3D%26quot%3Bline-height%3A%202%3B%20padding-left%3A%201em%3B%20text-indent%3A-1em%3B%26quot%3B%26gt%3B%5Cn%20%20%26lt%3Bdiv%20class%3D%26quot%3Bcsl-entry%26quot%3B%26gt%3BRayner%2C%20K.%20J.%2C%20Suarez%2C%20Y.%2C%20Davalos%2C%20A.%2C%20Parathath%2C%20S.%2C%20Fitzgerald%2C%20M.%20L.%2C%20Tamehiro%2C%20N.%2C%20Fisher%2C%20E.%20A.%2C%20Moore%2C%20K.%20J.%2C%20%26amp%3B%20Fernandez-Hernando%2C%20C.%20%282010%29.%20MiR-33%20Contributes%20to%20the%20Regulation%20of%20Cholesterol%20Homeostasis.%20%26lt%3Bi%26gt%3BScience%26lt%3B%5C%2Fi%26gt%3B%2C%20%26lt%3Bi%26gt%3B328%26lt%3B%5C%2Fi%26gt%3B%285985%29%2C%201570%26%23x2013%3B1573.%20%26lt%3Ba%20class%3D%26%23039%3Bzp-DOIURL%26%23039%3B%20href%3D%26%23039%3Bhttps%3A%5C%2F%5C%2Fdoi.org%5C%2F10.1126%5C%2Fscience.1189862%26%23039%3B%26gt%3Bhttps%3A%5C%2F%5C%2Fdoi.org%5C%2F10.1126%5C%2Fscience.1189862%26lt%3B%5C%2Fa%26gt%3B%26lt%3B%5C%2Fdiv%26gt%3B%5Cn%26lt%3B%5C%2Fdiv%26gt%3B%22%2C%22data%22%3A%7B%22itemType%22%3A%22journalArticle%22%2C%22title%22%3A%22MiR-33%20Contributes%20to%20the%20Regulation%20of%20Cholesterol%20Homeostasis%22%2C%22creators%22%3A%5B%7B%22creatorType%22%3A%22author%22%2C%22firstName%22%3A%22K.%20J.%22%2C%22lastName%22%3A%22Rayner%22%7D%2C%7B%22creatorType%22%3A%22author%22%2C%22firstName%22%3A%22Y.%22%2C%22lastName%22%3A%22Suarez%22%7D%2C%7B%22creatorType%22%3A%22author%22%2C%22firstName%22%3A%22A.%22%2C%22lastName%22%3A%22Davalos%22%7D%2C%7B%22creatorType%22%3A%22author%22%2C%22firstName%22%3A%22S.%22%2C%22lastName%22%3A%22Parathath%22%7D%2C%7B%22creatorType%22%3A%22author%22%2C%22firstName%22%3A%22M.%20L.%22%2C%22lastName%22%3A%22Fitzgerald%22%7D%2C%7B%22creatorType%22%3A%22author%22%2C%22firstName%22%3A%22N.%22%2C%22lastName%22%3A%22Tamehiro%22%7D%2C%7B%22creatorType%22%3A%22author%22%2C%22firstName%22%3A%22E.%20A.%22%2C%22lastName%22%3A%22Fisher%22%7D%2C%7B%22creatorType%22%3A%22author%22%2C%22firstName%22%3A%22K.%20J.%22%2C%22lastName%22%3A%22Moore%22%7D%2C%7B%22creatorType%22%3A%22author%22%2C%22firstName%22%3A%22C.%22%2C%22lastName%22%3A%22Fernandez-Hernando%22%7D%5D%2C%22abstractNote%22%3A%22%22%2C%22date%22%3A%222010-06-18%22%2C%22language%22%3A%22en%22%2C%22DOI%22%3A%2210.1126%5C%2Fscience.1189862%22%2C%22ISSN%22%3A%220036-8075%2C%201095-9203%22%2C%22url%22%3A%22http%3A%5C%2F%5C%2Fwww.sciencemag.org%5C%2Fcgi%5C%2Fdoi%5C%2F10.1126%5C%2Fscience.1189862%22%2C%22collections%22%3A%5B%22M2MNG549%22%5D%2C%22dateModified%22%3A%222015-07-27T14%3A34%3A20Z%22%7D%7D%2C%7B%22key%22%3A%22X9VF3HBH%22%2C%22library%22%3A%7B%22id%22%3A2474232%7D%2C%22meta%22%3A%7B%22creatorSummary%22%3A%22Lee%20et%20al.%22%2C%22parsedDate%22%3A%222010%22%2C%22numChildren%22%3A0%7D%2C%22bib%22%3A%22%26lt%3Bdiv%20class%3D%26quot%3Bcsl-bib-body%26quot%3B%20style%3D%26quot%3Bline-height%3A%202%3B%20padding-left%3A%201em%3B%20text-indent%3A-1em%3B%26quot%3B%26gt%3B%5Cn%20%20%26lt%3Bdiv%20class%3D%26quot%3Bcsl-entry%26quot%3B%26gt%3BLee%2C%20Y.%20W.%2C%20Lee%2C%20W.%20H.%2C%20%26amp%3B%20Kim%2C%20P.%20H.%20%282010%29.%20Role%20of%20NADPH%20oxidase%20in%20interleukin-4-induced%20monocyte%20chemoattractant%20protein-1%20expression%20in%20vascular%20endothelium.%20%26lt%3Bi%26gt%3BInflammation%20Research%26lt%3B%5C%2Fi%26gt%3B%2C%20%26lt%3Bi%26gt%3B59%26lt%3B%5C%2Fi%26gt%3B%289%29%2C%20755%26%23x2013%3B765.%20%26lt%3Ba%20class%3D%26%23039%3Bzp-DOIURL%26%23039%3B%20href%3D%26%23039%3Bhttps%3A%5C%2F%5C%2Fdoi.org%5C%2F10.1007%5C%2Fs00011-010-0187-3%26%23039%3B%26gt%3Bhttps%3A%5C%2F%5C%2Fdoi.org%5C%2F10.1007%5C%2Fs00011-010-0187-3%26lt%3B%5C%2Fa%26gt%3B%26lt%3B%5C%2Fdiv%26gt%3B%5Cn%26lt%3B%5C%2Fdiv%26gt%3B%22%2C%22data%22%3A%7B%22itemType%22%3A%22journalArticle%22%2C%22title%22%3A%22Role%20of%20NADPH%20oxidase%20in%20interleukin-4-induced%20monocyte%20chemoattractant%20protein-1%20expression%20in%20vascular%20endothelium%22%2C%22creators%22%3A%5B%7B%22creatorType%22%3A%22author%22%2C%22firstName%22%3A%22Yong%20Woo%22%2C%22lastName%22%3A%22Lee%22%7D%2C%7B%22creatorType%22%3A%22author%22%2C%22firstName%22%3A%22Won%20Hee%22%2C%22lastName%22%3A%22Lee%22%7D%2C%7B%22creatorType%22%3A%22author%22%2C%22firstName%22%3A%22Paul%20H.%22%2C%22lastName%22%3A%22Kim%22%7D%5D%2C%22abstractNote%22%3A%22%22%2C%22date%22%3A%229%5C%2F2010%22%2C%22language%22%3A%22en%22%2C%22DOI%22%3A%2210.1007%5C%2Fs00011-010-0187-3%22%2C%22ISSN%22%3A%221023-3830%2C%201420-908X%22%2C%22url%22%3A%22http%3A%5C%2F%5C%2Flink.springer.com%5C%2F10.1007%5C%2Fs00011-010-0187-3%22%2C%22collections%22%3A%5B%22M2MNG549%22%5D%2C%22dateModified%22%3A%222015-07-13T16%3A53%3A18Z%22%7D%7D%2C%7B%22key%22%3A%22H3XFQX7N%22%2C%22library%22%3A%7B%22id%22%3A2474232%7D%2C%22meta%22%3A%7B%22creatorSummary%22%3A%22Lee%20et%20al.%22%2C%22parsedDate%22%3A%222010%22%2C%22numChildren%22%3A0%7D%2C%22bib%22%3A%22%26lt%3Bdiv%20class%3D%26quot%3Bcsl-bib-body%26quot%3B%20style%3D%26quot%3Bline-height%3A%202%3B%20padding-left%3A%201em%3B%20text-indent%3A-1em%3B%26quot%3B%26gt%3B%5Cn%20%20%26lt%3Bdiv%20class%3D%26quot%3Bcsl-entry%26quot%3B%26gt%3BLee%2C%20Y.%20W.%2C%20Lee%2C%20W.%20H.%2C%20%26amp%3B%20Kim%2C%20P.%20H.%20%282010%29.%20Oxidative%20mechanisms%20of%20IL-4-induced%20IL-6%20expression%20in%20vascular%20endothelium.%20%26lt%3Bi%26gt%3BCytokine%26lt%3B%5C%2Fi%26gt%3B%2C%20%26lt%3Bi%26gt%3B49%26lt%3B%5C%2Fi%26gt%3B%281%29%2C%2073%26%23x2013%3B79.%20%26lt%3Ba%20class%3D%26%23039%3Bzp-DOIURL%26%23039%3B%20href%3D%26%23039%3Bhttps%3A%5C%2F%5C%2Fdoi.org%5C%2F10.1016%5C%2Fj.cyto.2009.08.009%26%23039%3B%26gt%3Bhttps%3A%5C%2F%5C%2Fdoi.org%5C%2F10.1016%5C%2Fj.cyto.2009.08.009%26lt%3B%5C%2Fa%26gt%3B%26lt%3B%5C%2Fdiv%26gt%3B%5Cn%26lt%3B%5C%2Fdiv%26gt%3B%22%2C%22data%22%3A%7B%22itemType%22%3A%22journalArticle%22%2C%22title%22%3A%22Oxidative%20mechanisms%20of%20IL-4-induced%20IL-6%20expression%20in%20vascular%20endothelium%22%2C%22creators%22%3A%5B%7B%22creatorType%22%3A%22author%22%2C%22firstName%22%3A%22Yong%20Woo%22%2C%22lastName%22%3A%22Lee%22%7D%2C%7B%22creatorType%22%3A%22author%22%2C%22firstName%22%3A%22Won%20Hee%22%2C%22lastName%22%3A%22Lee%22%7D%2C%7B%22creatorType%22%3A%22author%22%2C%22firstName%22%3A%22Paul%20H.%22%2C%22lastName%22%3A%22Kim%22%7D%5D%2C%22abstractNote%22%3A%22%22%2C%22date%22%3A%2201%5C%2F2010%22%2C%22language%22%3A%22en%22%2C%22DOI%22%3A%2210.1016%5C%2Fj.cyto.2009.08.009%22%2C%22ISSN%22%3A%2210434666%22%2C%22url%22%3A%22http%3A%5C%2F%5C%2Flinkinghub.elsevier.com%5C%2Fretrieve%5C%2Fpii%5C%2FS1043466609008114%22%2C%22collections%22%3A%5B%22M2MNG549%22%5D%2C%22dateModified%22%3A%222015-07-13T16%3A59%3A51Z%22%7D%7D%2C%7B%22key%22%3A%22B7KQ2KN3%22%2C%22library%22%3A%7B%22id%22%3A2474232%7D%2C%22meta%22%3A%7B%22creatorSummary%22%3A%22Hou%20et%20al.%22%2C%22parsedDate%22%3A%222008-04-14%22%2C%22numChildren%22%3A0%7D%2C%22bib%22%3A%22%26lt%3Bdiv%20class%3D%26quot%3Bcsl-bib-body%26quot%3B%20style%3D%26quot%3Bline-height%3A%202%3B%20padding-left%3A%201em%3B%20text-indent%3A-1em%3B%26quot%3B%26gt%3B%5Cn%20%20%26lt%3Bdiv%20class%3D%26quot%3Bcsl-entry%26quot%3B%26gt%3BHou%2C%20C.%20J.-Y.%2C%20Tsai%2C%20C.-H.%2C%20Su%2C%20C.-H.%2C%20Wu%2C%20Y.-J.%2C%20Chen%2C%20S.-J.%2C%20Chiu%2C%20J.-J.%2C%20Shiao%2C%20M.-S.%2C%20%26amp%3B%20Yeh%2C%20H.-I.%20%282008%29.%20Diabetes%20Reduces%20Aortic%20Endothelial%20Gap%20Junctions%20in%20ApoE-deficient%20Mice%3A%20Simvastatin%20Exacerbates%20the%20Reduction.%20%26lt%3Bi%26gt%3BJournal%20of%20Histochemistry%20and%20Cytochemistry%26lt%3B%5C%2Fi%26gt%3B%2C%20%26lt%3Bi%26gt%3B56%26lt%3B%5C%2Fi%26gt%3B%288%29%2C%20745%26%23x2013%3B752.%20%26lt%3Ba%20class%3D%26%23039%3Bzp-DOIURL%26%23039%3B%20href%3D%26%23039%3Bhttps%3A%5C%2F%5C%2Fdoi.org%5C%2F10.1369%5C%2Fjhc.2008.950816%26%23039%3B%26gt%3Bhttps%3A%5C%2F%5C%2Fdoi.org%5C%2F10.1369%5C%2Fjhc.2008.950816%26lt%3B%5C%2Fa%26gt%3B%26lt%3B%5C%2Fdiv%26gt%3B%5Cn%26lt%3B%5C%2Fdiv%26gt%3B%22%2C%22data%22%3A%7B%22itemType%22%3A%22journalArticle%22%2C%22title%22%3A%22Diabetes%20Reduces%20Aortic%20Endothelial%20Gap%20Junctions%20in%20ApoE-deficient%20Mice%3A%20Simvastatin%20Exacerbates%20the%20Reduction%22%2C%22creators%22%3A%5B%7B%22creatorType%22%3A%22author%22%2C%22firstName%22%3A%22C.%20J.-Y.%22%2C%22lastName%22%3A%22Hou%22%7D%2C%7B%22creatorType%22%3A%22author%22%2C%22firstName%22%3A%22C.-H.%22%2C%22lastName%22%3A%22Tsai%22%7D%2C%7B%22creatorType%22%3A%22author%22%2C%22firstName%22%3A%22C.-H.%22%2C%22lastName%22%3A%22Su%22%7D%2C%7B%22creatorType%22%3A%22author%22%2C%22firstName%22%3A%22Y.-J.%22%2C%22lastName%22%3A%22Wu%22%7D%2C%7B%22creatorType%22%3A%22author%22%2C%22firstName%22%3A%22S.-J.%22%2C%22lastName%22%3A%22Chen%22%7D%2C%7B%22creatorType%22%3A%22author%22%2C%22firstName%22%3A%22J.-J.%22%2C%22lastName%22%3A%22Chiu%22%7D%2C%7B%22creatorType%22%3A%22author%22%2C%22firstName%22%3A%22M.-S.%22%2C%22lastName%22%3A%22Shiao%22%7D%2C%7B%22creatorType%22%3A%22author%22%2C%22firstName%22%3A%22H.-I%22%2C%22lastName%22%3A%22Yeh%22%7D%5D%2C%22abstractNote%22%3A%22%22%2C%22date%22%3A%222008-04-14%22%2C%22language%22%3A%22en%22%2C%22DOI%22%3A%2210.1369%5C%2Fjhc.2008.950816%22%2C%22ISSN%22%3A%220022-1554%22%2C%22url%22%3A%22http%3A%5C%2F%5C%2Fjhc.sagepub.com%5C%2Flookup%5C%2Fdoi%5C%2F10.1369%5C%2Fjhc.2008.950816%22%2C%22collections%22%3A%5B%22M2MNG549%22%5D%2C%22dateModified%22%3A%222015-07-13T16%3A41%3A00Z%22%7D%7D%5D%7D
Papke, C. L., Tsunezumi, J., Ringuette, L.-J., Nagaoka, H., Terajima, M., Yamashiro, Y., Urquhart, G., Yamauchi, M., Davis, E. C., & Yanagisawa, H. (2015). Loss of fibulin-4 disrupts collagen synthesis and maturation: implications for pathology resulting from EFEMP2 mutations. Human Molecular Genetics, 24(20), 5867–5879. https://doi.org/10.1093/hmg/ddv308
Reho, J. J., Zheng, X., Asico, L. D., & Fisher, S. A. (2015). Redox signaling and splicing dependent change in myosin phosphatase underlie early versus late changes in NO vasodilator reserve in a mouse LPS model of sepsis. American Journal of Physiology - Heart and Circulatory Physiology, 308(9), H1039–H1050. https://doi.org/10.1152/ajpheart.00912.2014
Rotllan, N., Chamorro-Jorganes, A., Araldi, E., Wanschel, A. C., Aryal, B., Aranda, J. F., Goedeke, L., Salerno, A. G., Ramirez, C. M., Sessa, W. C., Suarez, Y., & Fernandez-Hernando, C. (2015). Hematopoietic Akt2 deficiency attenuates the progression of atherosclerosis. The FASEB Journal, 29(2), 597–610. https://doi.org/10.1096/fj.14-262097
Carlström, M., Liu, M., Yang, T., Zollbrecht, C., Huang, L., Peleli, M., Borniquel, S., Kishikawa, H., Hezel, M., Persson, A. E. G., Weitzberg, E., & Lundberg, J. O. (2015). Cross-talk Between Nitrate-Nitrite-NO and NO Synthase Pathways in Control of Vascular NO Homeostasis. Antioxidants & Redox Signaling, 23(4), 295–306. https://doi.org/10.1089/ars.2013.5481
Gao, N., Huang, J., He, W., Zhu, M., Kamm, K. E., & Stull, J. T. (2013). Signaling through Myosin Light Chain Kinase in Smooth Muscles. Journal of Biological Chemistry, 288(11), 7596–7605. https://doi.org/10.1074/jbc.M112.427112
Kuang, S.-Q., Kwartler, C. S., Byanova, K. L., Pham, J., Gong, L., Prakash, S. K., Huang, J., Kamm, K. E., Stull, J. T., Sweeney, H. L., & Milewicz, D. M. (2012). Rare, Nonsynonymous Variant in the Smooth Muscle-Specific Isoform of Myosin Heavy Chain, MYH11, R247C, Alters Force Generation in the Aorta and Phenotype of Smooth Muscle Cells. Circulation Research, 110(11), 1411–1422. https://doi.org/10.1161/CIRCRESAHA.111.261743
Fox, K. A., Longo, M., Tamayo, E., Gamble, P., Makhlouf, M., Mateus, J. F., & Saade, G. R. (2012). Sex-specific effects of nicotine exposure on developmental programming of blood pressure and vascular reactivity in the C57Bl/6J mouse. American Journal of Obstetrics and Gynecology, 207(3), 208.e1-208.e9. https://doi.org/10.1016/j.ajog.2012.06.021
Shai, S.-Y., Sukhanov, S., Higashi, Y., Vaughn, C., Rosen, C. J., & Delafontaine, P. (2011). Low circulating insulin-like growth factor I increases atherosclerosis in ApoE-deficient mice. AJP: Heart and Circulatory Physiology, 300(5), H1898–H1906. https://doi.org/10.1152/ajpheart.01081.2010
Fox, K. A., Longo, M., Tamayo, E., Kechichian, T., Bytautiene, E., Hankins, G. D. V., Saade, G. R., & Costantine, M. M. (2011). Effects of pravastatin on mediators of vascular function in a mouse model of soluble Fms-like tyrosine kinase-1–induced preeclampsia. American Journal of Obstetrics and Gynecology, 205(4), 366.e1-366.e5. https://doi.org/10.1016/j.ajog.2011.06.083
Rayner, K. J., Suarez, Y., Davalos, A., Parathath, S., Fitzgerald, M. L., Tamehiro, N., Fisher, E. A., Moore, K. J., & Fernandez-Hernando, C. (2010). MiR-33 Contributes to the Regulation of Cholesterol Homeostasis. Science, 328(5985), 1570–1573. https://doi.org/10.1126/science.1189862
Lee, Y. W., Lee, W. H., & Kim, P. H. (2010). Role of NADPH oxidase in interleukin-4-induced monocyte chemoattractant protein-1 expression in vascular endothelium. Inflammation Research, 59(9), 755–765. https://doi.org/10.1007/s00011-010-0187-3
Lee, Y. W., Lee, W. H., & Kim, P. H. (2010). Oxidative mechanisms of IL-4-induced IL-6 expression in vascular endothelium. Cytokine, 49(1), 73–79. https://doi.org/10.1016/j.cyto.2009.08.009
Hou, C. J.-Y., Tsai, C.-H., Su, C.-H., Wu, Y.-J., Chen, S.-J., Chiu, J.-J., Shiao, M.-S., & Yeh, H.-I. (2008). Diabetes Reduces Aortic Endothelial Gap Junctions in ApoE-deficient Mice: Simvastatin Exacerbates the Reduction. Journal of Histochemistry and Cytochemistry, 56(8), 745–752. https://doi.org/10.1369/jhc.2008.950816