Ideal for Eye Tissue Homogenization
Do you spend lots of time and effort homogenizing eye tissue samples? The Bullet Blender® tissue homogenizer delivers high quality and superior yields. No other homogenizer comes close to delivering the Bullet Blender’s winning combination of top-quality performance and budget-friendly affordability. See below for an eye tissue homogenization protocol.
The Bullet Blender® Homogenizer
Save Time, Effort and Get Superior Results
- Consistent and High Yield Results
Run up to 24 samples at the same time under microprocessor-controlled conditions, ensuring experimental reproducibility and high yield. Process samples from 10mg or less up to 3.5g. - No Cross Contamination
No part of the Bullet Blender® ever touches the eye tissue samples – the sample tubes are kept closed during homogenization. There are no probes to clean between samples. - Samples Stay Cool
Homogenizing causes only a few degrees of heating. Our Gold models keep samples at 4°C. - Easy and Convenient to Use
Just place beads and buffer along with your eye tissue sample in standard tubes, load tubes directly in the Bullet Blender, select time and speed, and press start. - Risk Free Purchase
The Bullet Blender® comes with a 30 day money back guarantee and a 2 year warranty, with a 3 year warranty on the motor. The simple, reliable design enables the Bullet Blenders to sell for a fraction of the price of ultrasonic or other agitation based instruments, yet provides an easier, quicker technique.
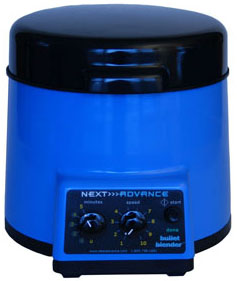
Bullet Blender Homogenization Protocol for Eye Tissue
Sample size |
See the Protocol |
microcentrifuge tube model (up to 300mg) | Small eye samples |
5mL tube model (100mg – 1g) | Medium eye samples |
50mL tube model (100mg – 3.5g) | Large eye samples |
Selected Publications for Eye Tissue
See all of our Bullet Blender publications!
Zhou, Y., Bennett, T. M., & Shiels, A. (2016). Lens ER-stress response during cataract development in Mip-mutant mice. Biochimica et Biophysica Acta (BBA) - Molecular Basis of Disease, 1862(8), 1433–1442. https://doi.org/10.1016/j.bbadis.2016.05.003
Charbel Issa, P., Barnard, A. R., Herrmann, P., Washington, I., & MacLaren, R. E. (2015). Rescue of the Stargardt phenotype in Abca4 knockout mice through inhibition of vitamin A dimerization. Proceedings of the National Academy of Sciences, 112(27), 8415–8420. https://doi.org/10.1073/pnas.1506960112
Doldur-Balli, F., Ozel, M. N., Gulsuner, S., Tekinay, A. B., Ozcelik, T., Konu, O., & Adams, M. M. (2015). Characterization of a novel zebrafish (Danio rerio) gene, wdr81, associated with cerebellar ataxia, mental retardation and dysequilibrium syndrome (CAMRQ). BMC Neuroscience, 16(1). https://doi.org/10.1186/s12868-015-0229-4
Can, N., Catak, O., Turgut, B., Demir, T., Ilhan, N., Kuloglu, T., & Ozercan, I. H. (2015). Neuroprotective and antioxidant effects of ghrelin in an experimental glaucoma model. Drug Design, Development and Therapy, 2819. https://doi.org/10.2147/DDDT.S83067
Eriksson, A., Williams, M. J., Voisin, S., Hansson, I., Krishnan, A., Philippot, G., Yamskova, O., Herisson, F. M., Dnyansagar, R., Moschonis, G., Manios, Y., Chrousos, G. P., Olszewski, P. K., Frediksson, R., & Schiöth, H. B. (2015). Implication of coronin 7 in body weight regulation in humans, mice and flies. BMC Neuroscience, 16(1), 13. https://doi.org/10.1186/s12868-015-0151-9
Patil, H., Saha, A., Senda, E., Cho, K., Haque, M., Yu, M., Qiu, S., Yoon, D., Hao, Y., Peachey, N. S., & Ferreira, P. A. (2014). Selective Impairment of a Subset of Ran-GTP-binding Domains of Ran-binding Protein 2 (Ranbp2) Suffices to Recapitulate the Degeneration of the Retinal Pigment Epithelium (RPE) Triggered by Ranbp2 Ablation. Journal of Biological Chemistry, 289(43), 29767–29789. https://doi.org/10.1074/jbc.M114.586834
Griffith, G. L., Kasus-Jacobi, A., Lerner, M. R., & Pereira, H. A. (2014). Corneal Wound Healing, a Newly Identified Function of CAP37, Is Mediated by Protein Kinase C Delta (PKCδ). Investigative Opthalmology & Visual Science, 55(8), 4886. https://doi.org/10.1167/iovs.14-14461
Lydic, T. A., Busik, J. V., & Reid, G. E. (2014). A monophasic extraction strategy for the simultaneous lipidome analysis of polar and nonpolar retina lipids. The Journal of Lipid Research, 55(8), 1797–1809. https://doi.org/10.1194/jlr.D050302
van der Plas-Duivesteijn, S. J., Mohammed, Y., Dalebout, H., Meijer, A., Botermans, A., Hoogendijk, J. L., Henneman, A. A., Deelder, A. M., Spaink, H. P., & Palmblad, M. (2014). Identifying Proteins in Zebrafish Embryos Using Spectral Libraries Generated from Dissected Adult Organs and Tissues. Journal of Proteome Research, 13(3), 1537–1544. https://doi.org/10.1021/pr4010585
Cho, K. -i., Patil, H., Senda, E., Wang, J., Yi, H., Qiu, S., Yoon, D., Yu, M., Orry, A., Peachey, N. S., & Ferreira, P. A. (2014). Differential Loss of Prolyl Isomerase or Chaperone Activity of Ran-binding Protein 2 (Ranbp2) Unveils Distinct Physiological Roles of Its Cyclophilin Domain in Proteostasis. Journal of Biological Chemistry, 289(8), 4600–4625. https://doi.org/10.1074/jbc.M113.538215
Aung, M. H., Park, H. n., Han, M. K., Obertone, T. S., Abey, J., Aseem, F., Thule, P. M., Iuvone, P. M., & Pardue, M. T. (2014). Dopamine Deficiency Contributes to Early Visual Dysfunction in a Rodent Model of Type 1 Diabetes. Journal of Neuroscience, 34(3), 726–736. https://doi.org/10.1523/JNEUROSCI.3483-13.2014
Cho, K., Haque, M., Wang, J., Yu, M., Hao, Y., Qiu, S., Pillai, I. C. L., Peachey, N. S., & Ferreira, P. A. (2013). Distinct and Atypical Intrinsic and Extrinsic Cell Death Pathways between Photoreceptor Cell Types upon Specific Ablation of Ranbp2 in Cone Photoreceptors. PLoS Genetics, 9(6), e1003555. https://doi.org/10.1371/journal.pgen.1003555
Mihai, D. M., Jiang, H., Blaner, W. S., Romanov, A., & Washington, I. (2013). The retina rapidly incorporates ingested C20-D₃-vitamin A in a swine model. Molecular Vision, 19, 1677–1683.